Cosmic Ray Spectral Studies
The spectrum of cosmic rays (CR) follows a power law with spectral index close to 2.7 for energies below 1014 eV, but it is considerably steeper for energies above 3 1015 eV. The observation of a change in spectral slope, or 'knee' in the fluxes of cosmic rays (Fig.1) at the energy scale 1015 eV has caused much speculation since its discovery over 40 years ago. The origin of this feature remains unknown, but it may suggest that more than one astrophysical process is responsible for cosmic ray acceleration. The bulk of CR are thought to be accelerated in galactic supernovae shocks. A class of models (e.g.: Lagage & Cesarsky, 1983) predict both a cutoff at an approximate maximum energy of Z x 1014 eV, where Z is the particle charge, and an elemental composition of primary cosmic rays shifting towards heavier elements at high energy.
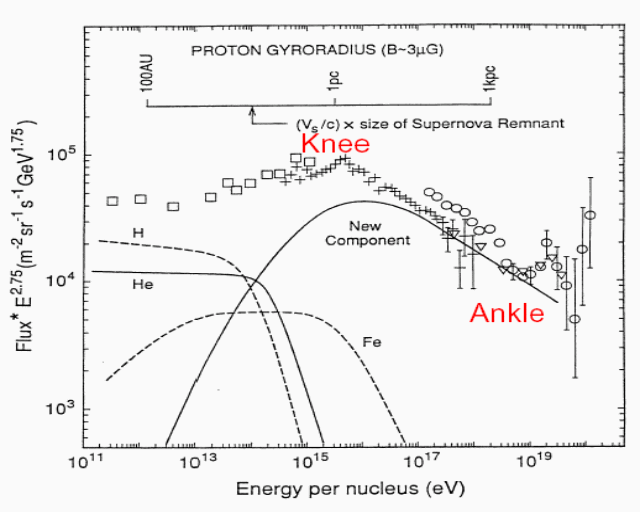
Fig. 1 : All-particle energy spectrum
- Direct measurements of primary cosmic ray energy spectra and of their elemental composition up to the highest energies are the goals of a series of experiments planned to take data in the next years. The aim is to understand the so far unknown mechanism of acceleration of primary cosmic rays of very high energy, to identify their sources and to clarify their interactions with the inter-galactic medium.
Measurements in this range of energies can be divided into two classes :
- indirect detection by ground-based air shower experiments.
- direct detection by balloon experiments (e.g.: JACEE , RUNJOB, ATIC, CREAM ) or by experiments in space (e.g.: the proposed experiment ACCESS on the International Space Station)
-
- Ground-based detectors observe the extensive showers of secondary particles initiated when a primary cosmic ray interacts with a nucleus of the upper atmosphere. The interpretation of air shower measurements is not straightforward and depends on assumptions regarding the nature of the particles that initiate the showers. Showers from heavier nuclei typically start higher in the atmosphere and develop more rapidly than showers from lighter nuclei. Estimates of the primary composition depend on parameters such as the depth of the shower maximum in the atmosphere and several other shower parameters, e.g.: muon content.
-
- The detection of cosmic rays above the atmosphere is the only way to obtain direct measurements of the primary particles and their energy spectra.
-
- Nuclear emulsions, interleaved with suitable converters, were used in JACEE and RUNJOB balloon flights to simultaneously measure the charge and energy of the primary particle. The payloads had to be recovered and the energy and charge information was extracted from the analysis of the emulsion data.
-
- In the next generation of direct experiments, data is recorded electronically in-flight and transmitted to the ground. The design of the instrument usually includes a combination of charge detection and energy measurements, with detectors such as scintillator hodoscopes, Silicon-pixel arrays combined with a Calorimeter or a Transition Radiation Detector (TRD).
-
- Limitations on the maximum allowed detector mass imposes severe constraints. Calorimeters deep enough to allow nearly full containment of hadron showers are simply too massive to be flown. A balloon-borne or space-based hadronic calorimeter should be deep enough in both interaction length and radiation length to generate interactions and contain e.m. showers produced by secondary neutral pions, be light enough to fly and yet retain an acceptable geometric factor.
A workable compromise is provided by thin calorimeters preceded by a light target (low-Z) consisting of a 0.5 to1.0 interaction length followed by a dense-absorber e.m. calorimeter at least 20 radiation lengths deep
-
- Transition radiation detectors rely on the passage of a particle through the detector without a nuclear interaction, however the limited number of radiators (usually constrained by the maximum allowed instrument size) has so far prevented their use for proton spectra measurements.
-
- The major limitation to all direct techniques to date is collection power. Due to the limited fluxes at high energy, large-acceptance detectors are required to collect a sufficiently large data sample within an acceptable length of time. This limit is constantly being pushed as with the recent advent of a new generation of balloons developed by NASA (ULDB) designed for 90 days flights and by constructing payloads that do not require pressurized vessels for operation.
As the highest energy achievable in a balloon experiment is determined by exposure, the balloon experiments that will dominate the scenario in the next years fall into two categories :
LDB : Long-Duration-Balloon (up to 3 weeks) flights (e.g.: ATIC experiment)
ULDB : Ultra-Long-Duration-Balloon (up to 3 months) flights (e.g.: CREAM experiment)
-
- The Advanced Thin Ionization Calorimeter (ATIC) Balloon Experiment is designed to measure the composition and energy spectra of cosmic rays from ~10 GeV to near 100 TeV utilizing a pixellated Si detector to determine the charge of the primary particle in conjunction with a scintillator hodoscope. Cosmic rays that interact in a Carbon target have their energy determined from the shower that develops within a fully active calorimeter composed of a stack of scintillating BGO crystals. ATIC's geometry factor is about 0.25 m2sr.
CREAM
Physics reach beyond 100 TeV will be an opportunity for CREAM designed to reach 500 TeV after a series of 3 Ultra Long Duration Balloon flights.
CREAM consists of a sampling Tungsten/Scintillating fiber calorimeter preceded by a graphite target with scintillator layers for trigger and track-reconstruction, a pixelated silicon charge detector (SCD), a transition radiation detector (TRD), and a segmented timing-based particle-charge detector (TDC). Simultaneous measurements of the energy and charge (Z) of a subset of nuclei by the complementary calorimeter (for Z >1) and TRD (for Z > 3) techniques, will allow in-flight inter-calibration of their energy scales. Multiple measurements of the charge of the primary particle will be carried out by the TCD, the fiber hodoscopes, and the silicon detector with pixel read-out to minimize the effect of backscatter from the calorimeter
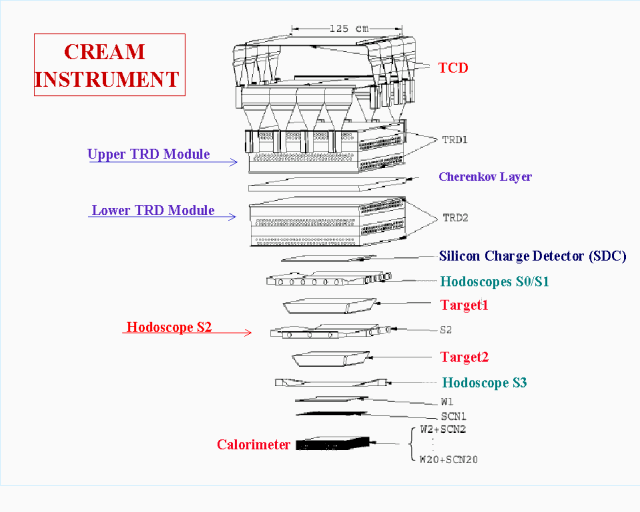
- CREAM's geometry factor is about 0.5 m2sr for protons. Effective exposure for heavy nuclei is greater than for protons due to their shorter interaction mean free path in the carbon target. The effective geometry factors for heavy nuclei is 1.3 m2sr approximately.
- Physics reach
The primary science objectives of the CREAM experiment are to:
- Determine whether or not the spectral slopes of the heavier nuclei are the same as that of Helium and different from that of protons;
-
- Search for spectral features, such as a cutoff in the proton spectrum.
-
- Measure potential changes in the spectra of secondary nuclei.
-
- The Italian institutions responsible for the research teams taking part in the CREAM mission are :
- The Italian funding agency providing support to the Italian institutions collaborating in the CREAM instrument is Istituto Nazionale di Fisica Nucleare (INFN).
-